Optimization and application of dry film photoresist for rapid fabrication of high-aspect-ratio microfluidic devices
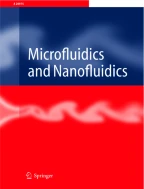
Fabrication of high-aspect-ratio PDMS microfluidic devices with conventional SU-8 based soft photolithography is challenging, and often, the thickness of the master from which PDMS replicas are molded is non-uniform. Here, we present an optimized, low cost, fast prototyping microfabrication technique to make deep (up to 500 μm) and high-aspect-ratio (up to 10) microfluidic channels by producing masters by laminating a single or multiple layers of a thin dry film photoresist onto metal wafers. In particular, we explore the required exposure energy for different film thicknesses as well as the highest achievable channel depths and aspect ratios. The homogeneity of the depth of PDMS channels formed using these masters is quantified and found to be remarkably uniform over distances of 20 mm or more. The importance of the processing parameters, such as the exposure energy and development time on final feature size, wall angle, and channel aspect ratio, is investigated. In addition, we report some failure cases, the potential reasons, and strategies for making optimized devices. Potentially, deep microfluidic channels with a wide range of aspect ratios can be used to make long, homogenous separation devices that can be used in cell sorting, filtration, and flow cytometry. We believe the protocols we outline here will be of great utility to the microfluidics community.
This is a preview of subscription content, log in via an institution to check access.
Access this article
Subscribe and save
Springer+ Basic
€32.70 /Month
- Get 10 units per month
- Download Article/Chapter or eBook
- 1 Unit = 1 Article or 1 Chapter
- Cancel anytime
Buy Now
Price includes VAT (France)
Instant access to the full article PDF.
Rent this article via DeepDyve
Similar content being viewed by others
Rapid prototyping of single-layer microfluidic PDMS devices with abrupt depth variations under non-clean-room conditions by using laser ablation and UV-curable polymer
Article 09 June 2017
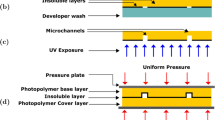
A mould-free soft-lithography approach for rapid, low-cost and bulk fabrication of microfluidic chips using photopolymer sheets
Article 10 October 2023
Soft lithography fabrication of index-matched microfluidic devices for reducing artifacts in fluorescence and quantitative phase imaging
Article 01 December 2017
Explore related subjects
References
- Carlier J et al (2004) Integrated microfluidics based on multi-layered SU-8 for mass spectrometry analysis. J Micromech Microeng 14:619. doi:10.1088/0960-1317/14/4/024ArticleGoogle Scholar
- Chen R-H, Cheng C-M (2001) Study of spin coating properties of SU-8 thick-layer photoresist. In: Advances in resist technology and processing XVIII, Proceedings of SPIE, Vol 4345
- Chen C-F, Gerlach TF (2013) Rapid prototyping of microfluidic modules with a water-developable dry-film photoresist bondable to PDMS. RSC Adv 3:14066–14072. doi:10.1039/C3RA41576GArticleGoogle Scholar
- Dahl JB, Lin J-MG, Muller SJ, Kumar S (2015) Microfluidic strategies for understanding the mechanics of cells and cell-mimetic systems. Annu Rev Chem Biomol 6:293–317. doi:10.1146/annurev-chembioeng-061114-123407ArticleGoogle Scholar
- del Campo A, Greiner C (2007) SU-8: a photoresist for high-aspect-ratio and 3D submicron lithography. J Micromech Microeng 17:R81–R95. doi:10.1088/0960-1317/17/6/R01ArticleGoogle Scholar
- Di Carlo D (2009) Inertial microfluidics. Lab Chip 9:3038–3046. doi:10.1039/B912547GArticleGoogle Scholar
- Faivre M, Abkarian M, Bickraj K, Stone HA (2006) Geometrical focusing of cells in a microfluidic device: an approach to separate blood plasma. Biorheology 43:147–159 Google Scholar
- Hansson J, Karlsson JM, Haraldsson T, Brismar H, van der Wijngaart W, Russom A (2012) Inertial microfluidics in parallel channels for high-throughput applications. Lab Chip 12:4644–4650. doi:10.1039/C2LC40241FArticleGoogle Scholar
- Hou HW, Bhagat AAS, Lin Chong AG, Mao P, Wei Tan KS, Han J, Lim CT (2010) Deformability based cell margination-A simple microfluidic design for malaria-infected erythrocyte separation. Lab Chip 10:2605–2613. doi:10.1039/C003873CArticleGoogle Scholar
- Hur SC, Tse HTK, Di Carlo D (2010) Sheathless inertial cell ordering for extreme throughput flow cytometry. Lab Chip 10:274–280. doi:10.1039/B919495AArticleGoogle Scholar
- Hur SC, Mach AJ, Di Carlo D (2011) High-throughput size-based rare cell enrichment using microscale vortices. Biomicrofluidics 5:022206. doi:10.1063/1.3576780ArticleGoogle Scholar
- Hur S, Brinckerhoff T, Walthers C, Dunn J, Di Carlo D (2012) Label-free enrichment of adrenal cortical progenitor cells using inertial microfluidics. PLOS One 7:e46550. doi:10.1371/journal.pone.0046550ArticleGoogle Scholar
- Kim HJ et al (2013) Disposable microfluidic blood cuvette for measuring hemoglobin concentration. Microsyst Technol 20:499–504. doi:10.1007/s00542-013-1954-1ArticleGoogle Scholar
- Lee MG, Shin JH, Bae CY, Choi S, Park J-K (2013) Label-free cancer cell separation from human whole blood using inertial microfluidics at low Shear stress. Anal Chem 85:6213–6218. doi:10.1021/ac4006149ArticleGoogle Scholar
- Leech PW, Wu N, Zhu Y (2009) Application of dry film resist in the fabrication of microfluidic chips for droplet generation. J Micromech Microeng 19:065019. doi:10.1088/0960-1317/19/6/065019(065016)ArticleGoogle Scholar
- Sollier E, Rostaing H, Pouteau P, Fouillet Y, Achard J-L (2009) Passive microfluidic devices for plasma extraction from whole human blood. Sens Actuators B 141:617–624. doi:10.1016/j.snb.2009.05.023ArticleGoogle Scholar
- Sollier E et al (2014) Size-selective collection of circulating tumor cells using Vortex technology. Lab Chip 14:63–77. doi:10.1039/C3LC50689DArticleGoogle Scholar
- Stephan K, Pittet P, Renaud L, Morin P, Ouaini N, Ferrigno R (2007) Fast prototyping using a dry film photoresist: microfabrication of soft-lithography masters for microfluidic structures. J Micromech Microeng 17:N69–N74. doi:10.1088/0960-1317/17/10/N01ArticleGoogle Scholar
- Stöhr U, Vulto P, Hoppe P, Urban G, Reinecke H (2008) High-resolution permanent photoresist laminate for microsystem applications. J Micro/Nanolithography MEMS MOEMS 7:033009. doi:10.1117/1.2964217ArticleGoogle Scholar
- Tsai Y-C, Jen H-P, Lin K-W, Hsieh Y-Z (2006) Fabrication of microfluidic devices using dry film photoresist for microchip capillary electrophoresis. J Chromatogr A 1111:267–271. doi:10.1016/j.chroma.2005.12.003ArticleGoogle Scholar
- Vulto P et al (2005) Microfluidic channel fabrication in dry film resist for production and prototyping of hybrid chips. Lab Chip 5:158–162. doi:10.1039/B411885EArticleGoogle Scholar
- Wang L et al (2016) Fast fabrication of microfluidic devices using a low-cost prototyping method. Microsyst Technol 22:677–686. doi:10.1007/s00542-015-2465-zArticleGoogle Scholar
- Xia Y, Whitesides GM (1998) Soft Lithography. Annu Rev Mater Sci 28:153–184. doi:10.1146/annurev.matsci.28.1.153ArticleGoogle Scholar
- Zhou J, Papautsky I (2013) Fundamentals of inertial focusing in microchannels. Lab Chip 13:1121–1132. doi:10.1039/C2LC41248AArticleGoogle Scholar
Acknowledgements
The authors would like to thank Prof. Dorian Liepmann (University of California Berkeley, SENSOR & ACTUATOR CENTER) for providing generous access to the profilometer in his lab. The first author appreciates financial support through the Postdoc fellowship from Fonds de recherche du Québec—Nature et Technologies (FQRNT). We are also grateful for partial financial support from The National Science Foundation (NSF, CBET-1066334).
Author information
Authors and Affiliations
- Department of Chemical and Biomolecular Engineering, University of California, Berkeley, CA, 94720, USA Fatemeh Khalkhal, Kendrick H. Chaney & Susan J. Muller
- Fatemeh Khalkhal